Evolution in road structural design
By Bob Humer, P.E.
From ancient times until the 1920s pavement structures were designed based on experience. Stone-paved streets originating 6,000 years ago in Mesopotamia (modern-day Iraq), were later followed by the Romans constructing more elaborate stone-paved roads with thick crushed base layers for drainage.
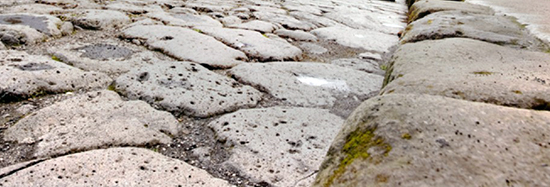
The first Macadam road was constructed in 1848 near Nottingham (UK) using coal tar, a waste product of coal gasification. Soon after, similar “tar concrete Macadam” projects followed in Paris (1854) and Washington D.C. (1871). From 1875 to 1900 Trinidad Lake Asphalt was extensively used worldwide as the binder in mixes. Still today it is sometimes used as an asphalt additive. In 1910 the City of Seattle specified the use of “natural bitumen or California oil asphalt” for their pavements.
Starting in the 1880s, asphalt binders were produced in California and later in Texas. By 1907 asphalt refined from crude oil surpassed the use of naturally occurring asphalts.
The concept of “what works, works” is to some extent still in practice today for less important city and county pavements.
Pioneers in the calculation of stresses and strains
The first attempts to calculate stresses and strains were for geotechnical problems of soil strength for earthen dams and foundations. However, no theoretical basis for soil foundation design was developed until the late 1700s. In 1717 the French engineer Henri Gautier recognized the “natural slope” of soils, later known as the soil’s “angle of repose” and a rudimentary soil classification was developed.
Later in 1773 engineer Charles Coulomb observed distinct slip planes in military ramparts and suggested that the maximum shear stress (τ) on the slip plane, for design purposes, was the sum of the soil cohesion, c, and friction, σ _tan Φ, where σ _is the normal stress on the slip plane and Φ _is the friction angle of the soil. By combining Coulomb’s theory with Christian Otto Mohr’s stress state, the theory became known as the Mohr-Coulomb theory.
By 1885, Joseph Boussinesq developed his original theory of stresses, strains, and deflections caused by a concentrated load applied to an elastic half-space (which can be integrated for a circular loaded area). Until Donald Burmister developed his layer theory in 1943, the Boussinesq solutions were the only ones available.
CBR method for pavement design
The California Bearing Ratio (CBR) procedure was first developed in 1929 by O.J. Porter of the California Highway Department. It is one of the oldest pavement design procedures. It is an empirical procedure relating the thickness of pavement required to the soaked CBR value of the subgrade. The CBR value is defined as the resistance to penetration of the subgrade soil relative to a standard crushed rock. The U.S. Army Corps of Engineers further developed the method and used it extensively for airfield and other pavements during and after the second world war. The method is applicable for multiple layers of successively higher CBR value material, as long as certain minimum layer thicknesses are adhered to.
The empirical approach to pavement structural design
Up to the 1930s, flexible pavements were designed by experience-based standards or catalogs. Once traffic loads and volumes increased significantly a more concerted effort was necessary to correlate pavement performance with pavement thickness, materials, subgrade, traffic and environmental factors. Thus, full-scale road tests started to be constructed, comprised of test sections for different combinations of materials, layer thicknesses and wheel loads.
Examples of such test roads are:
• Bates Road Test (IL), constructed in 1920 with 63 test sections comparing asphalt and concrete: the Chloe profilometer was introduced to measure the slope-variance representing roughness.
• Stockton Airfield Test (CA) conducted in 1948 by O.J. Porter for USACE, using accelerated pavement testing to verify Boussinesq calculations of pressures on soils under different aircraft gears and pavement structures.
• WASHO Road Test (ID), constructed in 1953 to determine the impact of heavy truck traffic on flexible pavements: featured the introduction of the Benkelman Beam, and the ESAL concept.
• Alconbury Hill Test (UK), constructed in 1957 (on the future A1) with 70 sections.
• AASHO Road Test (IL), launched in 1958, with a total of 836 segments in six test loops all built with different materials and structural layer thicknesses. The comprehensive project was the largest pavement research of the 20th century, and the results are still of importance in pavement design today.
• San Diego Test Road (CA), constructed in 1966, with 17 test sections, comparing Full-Depth vs. Conventional pavement structures.
• Westrack (NV), constructed in 1995 with 35 test sections, to study the effects of material differences, and to verify the SHRP prediction models and Level III mix design procedure.
• Long Term Pavement Performance (LTPP) General and Special Pavement Studies (GPS and SPS), with 2,180 test sections on existing roads (1989-2011), providing a very large database for pavement performance considering many design parameters.
• NCAT Test Track (AL), first constructed in 2000 with 46 sections to test experimental asphalt pavements. Accelerated heavy truck traffic is applied over a two-year period, after which the third year of the 3-year cycle is used for performance evaluations and construction and instrumentation for the next 3-year cycle.
Other test roads were built by Penn State (1970s), MN/ Road (1994-2014) and Ohio SHRP (1995-2016.
Accelerated loading facilities were built by; FHWA (VA), FAA (NJ), US ACEs (NH), Indiana (IN), UC/Berkeley/Davis (CA) and others around the world. Where the early tests produced a wealth of empirical data, the more recent pavement tests have been instrumented to measure stresses and strains to help bridge the gap between empiricism and mechanistic/analytical design methods. The results of all this expensive and time-consuming pavement research are shared throughout the world.
The Asphalt Institute’s “Thickness Design – Asphalt Pavements for Highways and Streets” manuals MS-1 (First edition, 1955) through MS-1 (Eight Edition), applied the results from these empirical tests. MS-1, 9th Edition (1999), is based on the multi-layer program DAMA. Already in the early 1960s, the Asphalt Institute Engineers concluded that bound asphalt layers contribute significantly more to pavement strength than aggregate base layers. This resulted in the Asphalt Institute registering the “Deep Strength (1965)”, “Deep-Lift” and “Full-Depth (1968)” Trademarks.
Mechanistic analysis
After Burmister developed his two and three-layer models, many other engineers and scientists (such as Terzaghi, Foster, Ahlvin, Ulery, Hveem, Porter, Jones, Peattie, Monismith, Nijboer, Huang, Lytton, Ullidtz, and many others) continued the development of the “homogeneous-isotropic-linear elastic multi-layer” models and their design parameters. Sven Nils Odemark (1949) developed a method for “Equivalent Thickness”, which facilitated early computer calculations.
Many refinements were later incorporated, such as visco-elasticity (Kelvin-Maxwell and Burgers models), temperature and moisture effects, wheel configurations and number of load positions.
By the late 1950s, the need for and interest in pavement design by more mechanistic/analytical methods resulted in the “First International Conference on the Design of Asphalt Pavements” (1962).
Conceived by Fred N. Finn (AI) and supported by Jess E. Buchanan (President of AI), this first conference brought the international pavement engineering community together and ignited the development of mechanistic/analytical tools for pavement structural design. Ten more such conferences have followed, organized by the “International Society for Asphalt Pavements” (ISAP).
Multi-layer elastic program
The first computer solution for a multi-layer system (CHEV5L) was presented by Chevron in 1963, followed by the program BISAR (Shell International). The Shell Asphalt Pavement Design Method was presented in 1977 at the “Fourth International Conference, Structural Design of Asphalt Pavements”.
The original limiting criteria in pavement structural design are:
• the cumulative vertical compressive strain at the top of the subgrade (to control rutting)
• the horizontal tensile strain at the bottom of the lowest bound layer (to control fatigue cracking from the bottom up).
For a single wheel load, the critical strain locations are centered below the load. For complex wheel configurations, such as with aircraft, the critical strain locations may be located elsewhere within the wheel assembly footprint depending on the pavement structure.
The rapid evolution in computer capabilities in the following decades resulted in many pavement design programs being developed with ever-increasing refinements for traffic, climate, materials, subgrade, construction quality, reliability, etc., as well as pavement performance data.
Examples of such multi-layer programs are:
• CHEV5L (1963), by Chevron (a.k.a. Chevron n-Layer)
• Bistro followed by BISAR (1977), by Shell International
• DAMA (1978), by Professor M. Witczak, and used for AI’s MS-1 (Roads) and MS-11 (Airports)
• DARWin, and DARWin-ME, (2008) by AASHTO
• Winjulia (JULIA), by USACE WES, used in WESLEA and LEDFAA
• KENLAYER, by Professor Yang H. Huang (University of Kentucky)
• Elsym5, by G. Ahlborn (UC Berkeley) and FHWA
• VESYS, by FHWA
The question remains: how many of these programs use the same core mechanistic algorithm? Besides the few programs listed here, many more have been developed over the years.
Basic input parameters are; E* modulus, Poisson’s ratio, layer thickness for each of the layers, traffic loads and frequency, and materials fatigue characteristics. Climate/environmental conditions, non-linear (viscoelastic) behavior, dynamic loading, and reliability are examples of further refinements.
Finite Element (FE) programs
Whereas multi-layer programs give a reasonable approximation of stresses and strains for assumed idealized conditions, finite element programs can give much more accurate solutions, especially when heterogeneous, anisotropic structures, viscoelastic and complex boundary conditions (such as crack discontinuities) need to be accounted for. Both axisymmetric and three-dimensional models are available, with the latter demanding significantly more computer time and capability.
The development of the finite element concept was first developed by Alexander Hrennikoff in 1941 and Richard Courant in 1943. In 1956 Ray Clough (Professor at UC Berkeley) co-authored one of the first papers on FE, first used the term “finite elements”, and developed one of the first FE programs (for Boeing and Bell Aerospace). A second Professor at UC Berkeley, Edward Wilson developed an FE program in the 1960s that has been widely used in civil, mechanical, and aeronautical engineering.
In FE programs, the structure is modeled with a mesh of discrete elements, represented by a node in each element’s center. Each node is assigned the individual element’s material properties (E*, Poisson’s ratio, etc.) while equilibrium equations represent the interactions between the nodes. With the displacements of all nodes known, the stresses and strains between all elements are then computed. The finer the mesh and the larger the number of elements, the better the accuracy of the structure’s simulation. For this reason, the use of high-capacity computers is required for FE Analysis.
Examples of FE programs used in pavement analysis, especially for nonlinear response characteristics of pavement materials, are ILLIPAVE, ABAQUS and FENLAP. The FE methodology is less often used due to its complexity, larger number of equilibrium equations and computer run-time required.
In a well-designed rut-resistant pavement, top-down cracking is most likely caused by the repeated shear stresses at the edge of the wheel loads (tires). Finite element programs can help determine the extent of these shear stresses at the tire’s edge.
Both multi-layer and FE programs are used to mechanistically calculate critical responses in the pavement (stress, strain, deformation). Combined with empirical field observations of performance (rutting, cracking as from Road Tests and Accelerated Loading Facilities) they result in what is called: “Mechanistic-Empirical design methods”.
And then “the world turned upside down”
Until recently, pavement structural design was focused on limiting the vertical subgrade compressive strain, and the horizontal tensile strain at the bottom of the bound layers. The fatigue characteristics of the lower lift of the bound layer in combination with the tensile strain and the number of load repetitions were used to control “bottom-up cracking” of the bound layer.
As traffic loads and volumes increased, new pavement and overlay designs resulted in thicker pavements to avoid this bottom-up cracking.
In the mid-1990s, pavement engineers started to notice that surface cracking in these thicker pavements started from the top and did not extend down more than approximately 4 inches, and that asphalt thicknesses larger than 8 to 9 inches did not increase pavement performance substantially.
This phenomenon required a re-thinking of the pavement design concepts. Not only can the commonplace mill-and-replace of the surface occur as needed, but also the bottom-up cracking can be avoided by limiting the strains in the lowest bound asphalt layer below what is called the endurance limit. It started the concept of Perpetual Pavement design.
As a result, pavement designs have become not thicker, but smarter. Now individual asphalt layers in the pavement structure are being designed with different mixes to accommodate the different stresses and strains each asphalt layer will experience.
The endurance limit (ε /ε max) is where a mix becomes independent of the number of load repetitions. Thus the structural design becomes only dependent on the magnitude of the single heaviest wheel load.
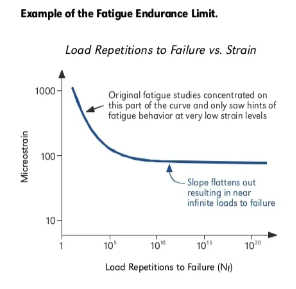
NCHRP Project 9-44 in conjunction with an international workshop defined the Fatigue Endurance Limit (FEL) as “a level of strain below which there is no cumulative damage over an infinite number of cycles.”
A significant number of research projects have resulted in the conclusion that the endurance limit is between conservatively 70 microstrain (1970, Monismith et al) and 125 microstrain (more typical), all depending on the mixes considered in the research. Shell International research in the 1960s already indicated that improved fatigue resistance of asphalt mixes is achieved at higher binder and lower air voids contents.
The Perpetual Pavement concept emerged from this understanding, consisting of:
(1) a 3-inch (75 mm) fatigue-resistant, asphalt-rich bottom layer
(2) an intermediate layer of highly rut-resistant asphalt mixture (variable thickness based on expected traffic loading); and
(3) a surface mixture layer of 3-4 inches (75- 100 mm) that may be periodically replaced as needed due to aging or environmental cracking without needing to replace or rehabilitate the intermediate and bottom layers of the pavement
The pavement structural design then varies the thickness of the intermediate rut-resistant lift, to keep the horizontal tensile strains in the bottom (three-inch) lift below the endurance limit.
The California-Caltrans Long-life (Perpetual Pavements) implementation
In 1996 Caltrans announced a new policy, to focus more on Long-life pavement designs. The first such project was developed as a perpetual pavement, in a combined effort by Caltrans, industry and academia. Construction started in 2002 on the southerly portion of I-710, in Los Angeles County. Seven more such long-life projects followed throughout the State, with the eighth one wrapping up construction in 2021. These projects all involved performance-related specifications and mix design test requirements. All but the first project have used intermediate layers with 25% RAP to increase stiffness.
In closing
Pavement designs are a complex problem and with all the sophistication and refinements, the calculated solutions remain an approximation. Using a particular software package without knowledge of the underlying theory and incorporated refinements can result in erroneous design recommendations. The use of two or three design methods for a particular project will give differing results, after which engineering judgment should be used to determine the final structural design recommendation.
Humer is an Asphalt Institute Senior Regional Engineer based in California
For a detailed study of Pavement Design, the following resources are recommended:
“Pavement Analysis and Design”, by Professor Yang M. Huang, of University of Kentucky, 1993.
“Principles of Pavement Design” (2nd Edition), by Professors E.J. Yoder and M.W. Witczak, 1975.
“Perpetual Asphalt Pavements, A Synthesis”, by D. Newcomb, R. Willis, and D. Timm, (APA) 2010
“Developments in Structural Design and Rehabilitation of Asphalt Pavements over Three-Quarters of a Century”, by Professors C.L. Monismith and S.F. Brown, AAPT Volume 68A, (pages 128 -251), 1999.